1.IntroductionNight-vision disturbances1 2 3 4 5 6 7 are frequent after different types of eye operations, such as refractive or cataract surgery (with mono- and multifocal intraocular lenses). Although the study and evaluation of night-vision disturbances began many decades ago,8 9 10 the recent growth of ocular surgery has given such studies special relevance. These disturbances, which include glare, starbursts, and halos, underlie some of the postoperative complaints, prompted by visual discomfort, especially under low illumination when the pupil is largest. The problem can even interfere with certain routine tasks, such as driving at night. Although the patient may report these disturbances, for example, in a questionnaire during a clinical visit, such information has serious limitations because subjective responses can be swayed by various psychological factors and may fail to reveal the magnitude of the problem, its evolution, or its relationship to other variables. Given the importance of ocular surgery, different tests are needed to quantify night-vision disturbances. It would facilitate the study of these phenomena, for example, to establish whether the subject suffers these disturbances, and if so, their magnitude and even the differences among subjects. It would also allow an exhaustive study of the relationships of these phenomena to different pathologies or to variables of the operation, thereby making monitoring easier. The development of devices for this purpose would therefore be useful for the clinician as well as the researcher concerned with these surgical operations. Here we present an experimental device that we call a halometer, which is designed to quantify the presence of halos in the vision of the subject. As is well known, a subject affected by halos sees rings around lights at night. Tests exist to evaluate glare, but it is rare to measure halos. In the test used for the latter, as described later, a subject, after dark adaptation, is asked to discriminate (detect) a small luminous source around a central high-luminance stimulus. Our device provides a disturbance index for evaluating the effect of halos on night vision. Laboratory comparisons of the results for normal subjects (control group) and others subjected to LASIK (laser in situ keratomileusis) surgery reveals that our experimental device is useful for analyzing and quantifying the phenomenon of halos. 2.Experimental Setup2.1.Description of the Test and Device (Halometer)The halometer consists basically of two boards within a methacrylate box (see Fig. 1). The two boards are connected by electrical wires fixed by screws. The front part of the box has a black cover also of methacrylate with holes drilled in it to permit the exit of light from LEDs situated on the board. The back part of the boards has guides and holes to isolate the LEDs. The electronic board is connected to the back part of the boards. The box is placed on a methacrylate bracket. A subject (Fig. 2) situated in front of the device would see a black screen with different holes where a central light source is surrounded by a series of luminous spots arranged in twelve radial lines. The central light source serves also to fix the observer’s gaze. The distance between the farthest spots of a line is 20.4 cm. The central spot has a diameter of 1.2 cm. In the experiments performed to check the device, the subjects were seated with the head supported by a chin and headrest 2 m from the device. The central and surrounding spots subtended 0.34 and 0.06 deg, respectively. Figure 2Front view of the device (halometer). The holes that permit the exit of light from the central and peripheral LEDs are viewed by the subject on a dark background. ![]() To simulate situations in which the subjects usually indicate halos, it is advisable to present stimuli with very high luminances. For this, the central source and the circular spot are GaN LEDs (large-emission diode) (LITE-ON Electronics Inc.) with a power of 120 mW and a direct current of 30 mA. The device is connected via a port to a personal computer (PC) that computerizes the data (Fig. 3). The luminance of the spots was calibrated with a Spectrascan Photoresearch PR-704 spectroradiometer. For this, we used two field sizes: for the central spot, a field of 0.125 deg was used since on providing very high luminances, it would saturate the spectroradiometer; for the peripherals, a field of 1 deg was used. The luminance of the spots was variable and controlled by a software program. The calibration of the device gave a luminance value for the central spot ranging from 2.75×103 cd/m 2 to 9.4×104 cd/m 2, while the luminance of the lateral circular spots ranged from 0.1 cd/m2 to 20.3 cd/m2. The luminance of the central spot allowed a broad margin for simulating situations of central sources with high luminance. For example, the luminance of the full moon is on the order of 2.5×103 cd/m 2, while that of the surface of a fluorescent lamp is 8×103 cd/m 2. It should be taken into account that, generally, devices such as color monitors (CRT or thin-film transistor types) do not reach high luminance maximums (averaging 350 cd/m2) and thus are not adequate for simulating these situations. The device also provides intermediate luminances for the central and lateral spots, which may be suitable for adjusting to the great range of the variables in this phenomenon: type of operation, degree of ametropia, age, etc. In the case of a subject who indicates strong glare during the experiment for a selected luminance, we would select a lower value. The task of the subject was to discriminate the lateral luminous spots with respect to the central spot. This test was chosen because a subject who sees halos around a central source should have greater difficulty in discriminating peripheral lights. For example, a refractive surgery patient is usually operated on within a central optical zone of 5 or 6 mm and a small additional transition zone of 1 to 2 mm. Under low illumination conditions, the pupil dilates and, depending on the observer, there would be an image on the retina generated by the central optical zone, another one by the transition zones, and even another by the unoperated anterior cornea. Since all these zones have different optical power, they form a defocused image with respect to that provided by the emmetropized central optical zone of the cornea. If the object has high luminance, the defocused zones can form halos that are perceptible to the observer. These halos would interfere with the discrimination of peripheral lights. It is important to point out that the phenomena of night-vision disturbances occur simultaneously rather than in an isolated way, and thus a subject that complains of halos may also perceive a certain glare in the image that also diminishes discrimination. 2.2.Determination of the Disturbance Index: Psychophysical Parameters and ProcedureDuring the experiments to determine the disturbance index, different methods11 12 and psychophysical parameters can be selected, depending on the object under study. When characterizing this device, we used a modified version of the constant-stimulus method. This method11 12 and its modifications are widespread and have been used in measuring different visual parameters.13 In the determination of the disturbance index, there are different phases, which are described in the following paragraphs. 2.2.1.Dark adaptationThis is needed to make the test measurements independent of the subject’s previous observation conditions and to simulate the most common situations in the appearance of night-vision disturbances. Thus an almost dark surrounding is used, in which the pupil size is large, corresponding to the scotopic or mesopic level. In our tests, we chose a dark-adaptation period of 3 min. Although total functional dark adaptation requires a longer period, different experimental data14 show that after the third minute the increase in the pupil size (the parameter we are interested in) is minimum for later times. In this way we ensured almost total dark adaptation, minimizing the duration of the experimental sessions to avoid tiring the observers because fatigue and distraction influence the data. 2.2.2.Central-stimulus adaptationAfter dark adaptation, the central stimulus is presented for 1 min before beginning the exposure to radial stimuli. In this phase, if the subject indicates that the luminance chosen for the central stimulus is very high, producing a high disability glare that impedes the execution of the experiment, another lower luminance is chosen, beginning again at the adaptation phase. 2.2.3.Stimulus presentation and detectionExposure time. We chose an exposure time of 1.25 s, which is similar to the value used in binocular-vision experiments with the modified-stimulus method.13 Although the eye under normal conditions can be stimulated with much shorter time values (∼0.001 s), the value selected is closer to the real situation, such as night vision, when the subject must discriminate or distinguish lights that are close to others that last for a longer time (not being quick flashes of light). On the other hand, a higher exposure value would excessively lengthen the experiment. For five normal observers, we also tested different exposure times of 0.75, 1, 1.25, and 1.5 s, without finding significant differences for the disturbance index. In such experiments, it might be useful for the stimulus to be presented more than once in the same session. Although it should be borne in mind that this option nevertheless has the limitation of lengthening the experiment, it is controllable by software, and a weight can be assigned to the stimulus, depending on the objective of the experiment. Time between stimuli. After the exposure of a stimulus, a time of 2 s was used without exposure to peripheral stimuli until the following stimulus appeared. During this time, only the central spot was presented. Learning effects. For five normal subjects and four surgical patients, we determined whether two prior sessions were sufficient to minimize the effects of learning and found that from the third session on, the disturbance index stabilized. Calculation of the disturbance index. This index is determined as the quotient of the area of the spots not detected by the subject, divided by the total area presented to the subject. It is expressed as a percentage. The higher the disturbance index, the lower the discrimination capacity, indicating a greater influence of halos. Selection of the area of the test presentation. The program permits selection of the area of stimuli to be presented to the subject. There are two options: to present the total stimulus are (twenty stimuli per diametrical line; see Fig. 2) which the device can present, or to restrict it to a smaller area (twelve stimuli) around the central stimulus. Presenting a smaller area offers the advantage of shortening the time of the sessions, and if the subject shows no additional anomaly, the stimuli with greater discrimination difficulty will be those surrounding the central stimulus. The choice of the larger area may be advisable for subjects who, owing to some abnormality or pathology, wish to know their peripheral-discrimination capacity or determine whether they have islands of vision. In addition, the program allows manual selection, enabling the subject to manipulate the program and device to present the stimuli along a particular line or within a given region. Sessions. A session is conducted as follows: After the adaptation period, the subject is presented with the stimuli completely at random, to avoid learning effects. On detecting peripheral spots the subject, presses a small remote control button, storing this information for subsequent treatment and for the calculation of the disturbance index. It bears pointing out that once an experimental session ends and before data are analyzed, the possible false alarms generated by the subject are checked to validate the experimental session or invalidate it and call for repetition. 3.Checking the Device: Experiments for Control Subjects and Surgical PatientsA key issue in corroborating the validity of the device is to test whether the parameter used to evaluate the halos (disturbance index) can discriminate between subjects who are affected by halos and those who are not. For this, we determined the disturbance index with emmetropic and ametropic subjects who were corrected but not subjected to surgery and who presented no visual pathology that might deteriorate their vision (control group), and we also calculated the disturbance index for surgical patients. The control group was made up of 40 subjects (23 males, 17 females; 22 ametropic corrected and 18 emmetropic). A total of 34 patients (19 females, 15 males) had undergone refractive surgery by LASIK (optical zone, 6 mm; up to 7.5 mm transition zones) in a clinic specializing in refractive surgery using the Esiris scanning spot excimer laser (Schwind). The age of the surgical patients ranged from 22 to 47 years. Their mean preoperative spherical refractive error was −4.6±2.2 D (standard deviation), ranging from −2 to −7.5 D. No subject had any previous astigmatism exceeding ±0.5 D. Given the interest of our study, we selected surgical patients for whom we could consider the operation satisfactory (emmetropic), because if it was not, our objective would be defeated by having begun with a sample of patients whose surgery was unsatisfactory. Therefore the patients fulfilled the following conditions: after three months, they were satisfied with the outcome of the surgery, no longer used any form of optical correction, and their mean postoperative spherical refractive error did not exceed 0.5 D. Despite these conditions, after surgery, 28 patients reported certain difficulties (to a greater or lesser degree) in night vision, including halos, as described in the literature. Six surgical patients did not claim any difficulties but performed the experiment because, although they were not conscious of it, their discrimination capacity could have been diminished. For 22 of the 34 patients, we were also able to conduct the presurgery experiment with their best correction. The best correction of these patients involved glasses (12 patients) or monocular contact lens (10 patients). None of them used bifocal contact lenses, which, being a multifocal system, could generate unfocused images that would invalidate the experiment.15 Of these 22 patients, 4 did not complain of halos. As indicated earlier, all the subjects who participated in the study underwent two prior tests to minimize learning effects. The test was administered in a dark room with only the illumination of the computer screen where the experiments were presented. The luminance of this screen was very dim and the screen was separated from the zone of the experiments by a black cardboard partition so that the light did not reach the observer directly. The experimental conditions under which the tests were made were 3 min of dark adaptation and 1 min of adaptation to the central stimulus. In additional tests for 5 control subjects and 4 surgical patients, it was confirmed that it was not necessary to use the total area of the device since they easily discriminated the peripheral stimuli. Thus, for all the subjects, we selected the near area (twelve stimuli per line), where discrimination difficulties that are due to halos as well as significant differences between normal subjects and surgical patients arise. We made three measurements of the disturbance index for each subject (patients and control group) and each luminance combination tested. We took four luminance combinations, two values for the central stimulus (3×103 cd/m 2 and 104 cd/m 2) and two for the lateral spots (0.75 and 4 cd/m2). The reasons for selecting these combinations were as follows: The luminance values used for the central spot guaranteed a value (of the same magnitude as and greater than that given by the full moon) that could simulate intense or brilliant lights, for example, in a nighttime task such as driving. In all subjects (patients and control group), we found that this value did not cause glare to impede observer performance. For the lateral spots, the values chosen corresponded to much lower luminance values (on the limit between mesopic and photopic conditions), the discrimination of which was more difficult owing to the possible halo generated by the intense light, but with a luminance value perceptible under normal conditions and one that enabled the quantification of the effect of halos. Table 1 shows the average results for the control subjects and the surgical patients, using two luminance levels for the central stimulus and two levels for the peripheral spots. The main result was that significant differences were found (P<0.05 according to Student’s t-test) among the averages of the control group and LASIK patients in all the experimental cases studied. The average values for the two groups did not depend on the intensity of the central source for the two values tested, and no significant differences (P>0.05) were found for the luminance values 3×103 cd/m 2 or 104 cd/m 2. Minor differences were found when the intensity of the peripheral stimuli was varied, but in no case reached statistical significance (P>0.05). The results, according to our experimental device and proposed disturbance index, indicate that the discrimination capacity under dark adaptation diminished for surgical patients with respect to the normal subjects, as reported in the literature.1 3 5 6 In addition, studying the variation range of each group, we found that the higher disturbance index for a nonsurgical subject (33.2±0.8) was significantly lower (P<0.0001) than the best index for a LASIK patient (39.2±0.9). Table 1
We found no significant difference (P>0.05) for the disturbance index between ammetropic corrected and emmetropic subjects in the control group for all the combinations of luminance tested. The average of the disturbance index for these luminance combinations is shown in Table 2. No significant presurgery differences were found in the disturbance index according to the type of correction (contact or spectacle lenses) for the surgical patients (see Table 2). Nor was any significant difference found that was due to gender. Table 2
An important issue was to ascertain whether the 22 surgical patients for whom we were able to take data prior to surgery underwent diminished discrimination capacity after surgery. For each of the 22 patients (including 4 who did not mention halos), with the four luminance combinations tested, the average for the disturbance index after surgery was significantly higher (P<0.05). The average values (see Table 2) for these 22 subjects were 26.1±2.1 and 52.8±3.3 before and after surgery, respectively, these values differing significantly (P<0.0001). These results agree with the subjective evaluation provided by the patients concerning the halos and even showed the high sensitivity of the test in detecting poorer discrimination in night vision among patients who did not even note the halo phenomenon. Nevertheless, it should be indicated that these results are limited by the fact that we had presurgery data for only 4 patients, who furthermore did not complain about problems of halos. In summary, this experimental device (halometer) can accurately evaluate the influence of halos, these being frequent symptoms in subjective descriptions given by patients after refractive surgery or cataract operations. Given the quantitative index provided, the device enables a comparison of pre- as well as postsurgical discrimination capacity under low illumination. This makes it possible to study the temporal evolution of the phenomenon of halos and also enables halos to be numerically correlated with different variables (age, type of eye operation, possible pathologies) that are usually relevant in different surgical contexts. REFERENCES
M. Pop
and
Y. Payette
,
“Photorefractive keratectomy versus laser in situ keratomileusis: a control-matched study,”
Ophthalmology , 107 251
–257
(2000). Google Scholar
L. Lesueur
,
B. Gajan
,
M. Nardin
,
E. Chapotot
, and
J. L. Arne
,
“Comparison of visual results and quality of vision between two multifocal intraocular lenses. Multifocal silicone and bifocal PMMA,”
J. Fr. Ophtalmol. , 23 355
–359
(2000). Google Scholar
G. Lafond
,
“Treatment of halos after photorefractive keratectomy,”
J. Refract. Surg. , 13 340
(1997). Google Scholar
D. K. Holmes-Higgin
and
T. E. Burris
,
“Corneal surface topography and associated visual performance with INTACS for myopia: phase III clinical trial results. The INTACS Study Group,”
Ophthalmology , 107 2061
–2071
(2000). Google Scholar
T. Seiler
,
W. Reckmann
, and
R. K. Maloney
,
“Effective spherical aberration of the cornea as a quantitative descriptor in corneal topography,”
J. Cataract Refractive Surg. , 19 155
–165S
(1993). Google Scholar
W. W. Haw
and
E. E. Manche
,
“Effect of preoperative pupil measurements on glare, halos, and visual function after photoastigmatic refractive keratectomy,”
J. Cataract Refractive Surg. , 27 907
–916
(2001). Google Scholar
C. R. Munnerly
,
S. J. Koons
, and
J. Marshall
,
“Photorefractive keratectomy: a technique for laser refractive surgery,”
J. Cataract Refractive Surg. , 14 46
–51
(1988). Google Scholar
H. H. Emsley
and
E. F. Fincham
,
“Diffraction halos in normal and glaucomatous eyes,”
Trans. Opt. Soc., London , 23 225
–240
(1922). Google Scholar
G. C. Simpson
,
“Ocular halos and coronas,”
Br. J. Ophthamol. , 37 450
–486
(1953). Google Scholar
J. Mellerio
and
D. A. Palmer
,
“Entoptic halos,”
Vision Res. , 10 595
–599
(1970). Google Scholar
J. R. Jime´nez
,
M. Rubin˜o
,
L. Jime´nez del Barco
, and
E. Hita
,
“Influence of the luminance and opponent chromatic channels on stereopsis with random-dot stereograms,”
Vision Res. , 37 591
–596
(1997). Google Scholar
P. Reeves
,
“The response of the average pupil to various intensities of light,”
J. Opt. Soc. Am. , 4 35
(1920). Google Scholar
J. R. Jime´nez
,
J. J. Durba´n
, and
R. G. Anera
,
“Testing Acuvue bifocal contact lens for maximum disparity with changes in illumination,”
Optom. Vision Sci. , 79 170
–174
(2002). Google Scholar
|
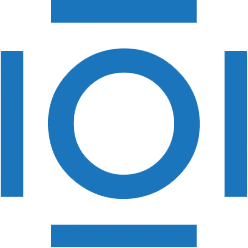
CITATIONS
Cited by 42 scholarly publications.
Surgery
LASIK
Laser therapeutics
Night vision
Contact lenses
Control systems
Light emitting diodes