|
Library of Congress Preassigned Control Number: 2015931195 for Field Guide to Lidar (ISBN 9781628416541). Published by SPIE P.O. Box 10 Bellingham, Washington 98227-0010 USA Phone: +1.360.676.3290 Fax: +1.360.647.1445 Email: books@spie.org Web: http://spie.org The content of this book reflects the work and thought of the author. Every effort has been made to publish reliable and accurate information herein, but the publisher is not responsible for the validity of the information or for any outcomes resulting from reliance thereon. Printed in the United States of America. First printing Introduction to the SeriesWelcome to the SPIE Field Guides—a series of publications written directly for the practicing engineer or scientist. Many textbooks and professional reference books cover optical principles and techniques in depth. The aim of the SPIE Field Guides is to distill this information, providing readers with a handy desk or briefcase reference that provides basic, essential information about optical principles, techniques, or phenomena, including definitions and descriptions, key equations, illustrations, application examples, design considerations, and additional resources. A significant effort will be made to provide a consistent notation and style between volumes in the series. Each SPIE Field Guide addresses a major field of optical science and technology. The concept of these Field Guides is a format-intensive presentation based on figures and equations supplemented by concise explanations. In most cases, this modular approach places a single topic on a page, and provides full coverage of that topic on that page. Highlights, insights, and rules of thumb are displayed in sidebars to the main text. The appendices at the end of each Field Guide provide additional information such as related material outside the main scope of the volume, key mathematical relationships, and alternative methods. While complete in their coverage, the concise presentation may not be appropriate for those new to the field. The SPIE Field Guides are intended to be living documents. The modular page-based presentation format allows them to be updated and expanded. We are interested in your suggestions for new Field Guide topics as well as what material should be added to an individual volume to make these Field Guides more useful to you. Please contact us at fieldguides@SPIE.org. John E. Greivenkamp, Series Editor College of Optical Sciences The University of Arizona The Field Guide SeriesKeep information at your fingertips with the SPIE Field Guides:
Field Guide to LidarThis Field Guide covers active electro-optical sensing, in which a sensor sends out a laser pulse and then measures the parameters of the return signal. Various groups refer to this type of sensor as a ladar, lidar, LIDAR, LADAR, or laser radar. For simplicity, only the term lidar is used throughout this book. The book is presented from the perspective of a lidar engineer. It covers a wide breadth, from simple 2D direct-detection lidars to multiple subaperture synthetic aperture lidars. It also covers a broad range of objects to be viewed, and distances from which to view the objects. Lasers and modulation are discussed in the context of their use in lidars. Other topics covered include receivers, apertures, and atmospheric effects in the context of lidar use and design. All lidars will be limited by the media between the lidar and the target, but atmospheric compensation techniques can often mitigate this limitation. These limitations and compensation approaches are presented. Many types of lidars are included along with appropriate data processing techniques. The lidar range equation in its many variations is discussed along with receiver noise issues that determine how much signal must be received to detect an object. This Field Guide is a handy reference to quickly access information on any aspect of lidars. It will be useful to students and lidar scientists or engineers who need an occasional reminder of the correct approaches or equations to use in certain applications. It will also be useful to systems engineers gaining a perspective on this rapidly growing technology. Paul McManamon March 2015 Table of ContentsGlossary of Symbols and Acronyms x Introduction 1 Introduction 1 Terms for Active Electro-optic Sensing 2 Types of Lidars 3 Lidars for Surface-Scattering (Hard) Targets 4 Lidars for Volume-Scattering (Soft) Targets 5 History of Lidar 6 Lidar Detection Modes 7 Spatial Coherence 8 Temporal Coherence 9 Eye Safety Considerations 10 Laser Safety Categories 11 Monostatic versus Bistatic Lidar 12 Transmit/Receive Isolation 13 Lidar Range Equation 14 Lidar Range Equation 14 Lidar Cross Section 15 Cross Section of a Corner Cube 16 Speckle 17 Atmospheric Absorption 18 Atmospheric Scattering 19 Atmospheric Turbulence 20 Aero-optical Effects on Lidar 21 Extended (Deep) Turbulence 22 Laser Power for Lidar 23 Lidar Signal-to-Noise Ratio 24 Direct Detection Signal-to-Noise Ratio 25 Noise Probability Density Functions 26 Thermal Noise 27 Shot Noise 28 The Sun as Background Noise 29 Dark Current, 1/f, and Excess Noise 30 Avalanche Photodiodes and Direct Detection 31 Number of Photons Required for a GMAPD Lidar Camera 32 Heterodyne Detection 33 Temporal Heterodyne Detection 34 Heterodyne Mixing Efficiency 35 Quadrature Detection 36 Carrier-to-Noise Ratio for Temporal Heterodyne Detection 37 Spatial Heterodyne Detection/Digital Holography 38 SNR for Spatial Heterodyne Detection 39 Types of Lidars 40 1D Range-Only Lidar 40 Tomographic Imaging Lidar 41 Range-Gated Active Imaging (2D Lidar) 42 3D Scanning Lidar 43 3D Flash Imaging 44 Geiger-Mode APD Flash Lidar 45 Linear-Mode APD Flash Lidar 46 Polarization-based Flash Lidar using Framing Cameras 47 Laser Vibration Detection 48 Synthetic Aperture Lidar 49 Inverse Synthetic Aperture Lidar 50 Range Doppler Imaging Lidar 51 Laser-Induced Breakdown Spectroscopy 52 Laser-Induced Fluorescence Lidar 53 Active Multispectral Lidar 54 Lidars Using Polarization as a Discriminant 55 Speckle Imaging Lidar 56 Phased Array of Phased-Array Imaging Lidar 57 Multiple Subapertures on Receive for Lidar 58 Multiple-Input, Multiple-Output Lidar 59 Methods of Phasing MIMO Lidars 60 Lidar Sources and Modulations 61 Lidar Sources and Modulations 61 Laser Resonators 62 Three-Level and Four-Level Lasers 63 Bulk Solid State Lasers for Lidar 64 Fiber Lasers for Lidar 65 Higher-Peak-Power Waveguide Lasers for Lidar 66 Diode Lasers for Lidar 67 Quantum Cascade Lasers for Lidar 68 Laser Pumping Considerations 69 Nonlinear Devices to Change the Lidar Wavelength 70 Q-Switched Lasers for Lidar 71 Pockels Cells 72 Mode-Locked Lasers for Lidar 73 Laser Seeding for Lidar 74 Laser Amplifiers for Lidar 75 Multiple Coherent Laser Transmitters 76 Laser Waveforms for Lidar 77 Polypulse Laser Waveforms 78 Linear Frequency Modulation for Lidar 79 Pseudo-random-Coded Lidar 80 RF Modulation of a Direct Detection Lidar 81 Lidar Receivers 82 Linear-Mode APD Arrays for Lidar 82 Geiger-Mode APD Arrays for Lidar 83 Receivers for Coherent Lidars 84 Acousto-optic Frequency Shifting 85 Long-Frame-Time Framing Detectors for Lidar 86 Gated Framing Cameras for 2D Lidar Imaging 87 Lidar Image Stabilization 88 Range Resolution of Lidar 89 Velocity Resolution of Lidar 90 Unambiguous Range 91 Point Spread Function 92 Beam Steering for Lidars 93 Gimbals for Use with Lidar 93 Fast-Steering Mirrors 94 Risley Prisms and Gratings 95 Rotating Polygonal Mirrors 96 Modulo 2π Beam Steering 97 Largest Steering Angle for an Optical Phased Array 98 Liquid Crystal Optical Phased Arrays 99 LC Fringing-Field Effect on Steering Efficiency 100 Reduction in Steering Efficiency Due to Quantization 101 Chip-Scale Optical Phased Arrays 102 MEMS Beam Steering for Lidar 103 Electrowetting Beam Steering for Lidar 104 Steerable Electro-evanescent Optical Refractors 105 Electro-optical Effects 106 Polarization Birefringent Grating Beam Steering 107 Step Angle Steering with LC Polarization Gratings 108 Multiple-Stage LCPGs 109 Lenslet-based Beam Steering 110 Electronically Written Lenslets 111 Mixed-Lenslet Arrays 112 Holographic Gratings for Beam Steering 113 Geometrical Optics 114 Lidar Processing 115 Inertial Measurement Units 115 Microscanning of Lidar Images for Improved Sampling 116 Range Measurement Processing 117 Nyquist Sampling a Range Profile 118 Threshold, Leading Edge, and Peak Detectors 119 Range Resolution, Precision, and Accuracy 120 Fourier Transforms 121 Developing 3D Maps from Lidar 122 3D Metrics for Lidar Images 123 Multiple-Subaperture Spatial Heterodyne Processing 124 Definitions of Lidar Data Processing Stages 125 Processing Laser Vibrometry Data 126 Target Classification Using Lidar 127 Equation Summary 128 Figure Sources 138 Bibliography 141 Index 143 Glossary of Symbols and Acronymsa amplitude of the (super) Gaussian A length of one side of a tetrahedral Aillum area illuminated by the transmitter AO acousto-optic AOM acousto-optic modulator Ap area of the pixel at the target location APD avalanche photodiode APS active-pixel sensor Arec area of the receiver aperture b zero position, or offset, of the (super) Gaussian beam B bandwidth c Gaussian, or super-Gaussian, beam width c speed of light cw continuous wave Cl coherence length CCD charge-coupled device CDMA code-division multiple access CMOS complementary metal-oxide semiconductor CNR carrier-to-noise ratio d cross-range resolution d required lens thickness d width of the individual radiator or receiver D aperture diameter DAiry diameter out to the zeros of the diffraction-limited spot at the focus for a circular aperture DAS detector angular subtense DFLC dual-frequency liquid crystal DIAL differential absorption lidar DM deformable mirror DOP degree of polarization e charge on an electron E energy at range E0 initial energy before traveling through the atmosphere EBAPS® electron-bombarded active-pixel sensor EBS electron-bombarded semiconductor Ein input electric field into a Jones matrix ELO local oscillator field EM electromagnetic EO electro-optic Eout input electric field into a Jones matrix Ep energy in a photon ER received energy per pulse Esig returned signal field ET transmitted energy per pulse Eth thermal energy Exin x portion of the input electric field Exout x portion of the output electric field Eyin y portion of the input electric field Eyout y portion of the output electric field f focal length of the lens f/# F-number of an optical element fl focal length of a lenslet f(x) Gaussian or super-Gaussian beam profile in one dimension F excess noise factor associated with the preamplifier gain FDMA frequency-division multiple access FFT fast Fourier transform FLC ferroelectric liquid crystal FLIR forward-looking infrared (camera) FM frequency modulated FOV field of view FPA focal plane array FSM fast-steering mirror G avalanche gain GIQE general image quality equation GMAPD Geiger-mode avalanche photodiode GML Geiger-mode lidar h Planck’s constant HWP half-wave plate ibk background current idk dark current in noise current in the detector is signal current in the detector ishotLO shot noise from the local oscillator ishot,sig shot noise from the signal ith thermal noise current I intensity of the beat between the local oscillator and the return signal Idkb bulk dark current Idks surface dark current IF intermediate frequency IMU inertial measurement unit IR infrared k effective elastic constant k number of photons in M events k Boltzmann constant L distance flown L length of the laser cavity LCPG liquid crystal polarization grating LFM linear frequency modulation LIBS laser-induced breakdown spectroscopy LIF laser-induced fluorescence LIMAR laser imaging and ranging LMAPD linear-mode avalanche photodiode LO laser oscillator LWIR long-wave infrared Lλ radiance per wavelength M number of events M2 measure of the spatial coherence of a laser beam. An M2 of 1 means it is diffraction limited. MEMS micro-electro-mechanical system MIMO multiple input, multiple output MO master oscillator MPE maximum permissible exposure MWIR midwave infrared n index of refraction n number of individual radiators or receivers nm diffraction efficiency of the mth order N number of photons per pixel received during a measurement time N super-Gaussian beam number. Higher numbers mean a more flat-topped beam shape. NA numerical aperture NEPh noise-equivalent photons NIIRS National Imagery Interpretability Rating Scale NIR near infrared OPA optical parametric amplifier OPA optical phased array OPD optical path difference OPO optical parametric oscillator p(k) Gaussian probability distribution P number of modes PAPA phased array of phased arrays PLO local oscillator power PPLN periodically poled lithium niobate PS signal power received PSD power spectral density PSF point spread function PT power transmitted Pthdbm thermal noise power q Poisson distribution probability q number of discrete steps QCL quantum cascade laser QWP quarter-wave plate r0 Fried parameter R range to the target
detector responsivity RF radio frequency RL load resistance ROIC readout integrated circuit Runambig unambiguous range S′3 = S3/S0 normalized Stokes parameter corresponding to ellipticity of incident light SNR signal-to-noise ratio SPGD stochastic parallel gradient descent SS solid state SWIR short-wave infrared t cell thickness tlens(waz, wel) lens phase profile T temperature T time separation between pulses TDMA time-division multiple access Tm time period over which a measurement is made v velocity of the lidar with respect to the surrounding air V platform velocity V relative velocity between the lidar and the target V voltage on an electrode VCSEL vertical-cavity surface-emitting laser Vt threshold voltage
beam width in azimuth and elevation for a Gaussian profile β angle between the slow axis of the half-wave plate and the x axis in the Jones matrix β atmospheric decay constant γ viscosity Δf change in frequency due to the Doppler shift Δn change in index of refraction Δz surface roughness ΔR range resolution Δt mode-locked pulse width ΔV velocity resolution Δx lenslet motion
angular resolution for a synthetic aperture lidar Δλ linewidth of the laser in wavelength
angular motion used in an inverse synthetic aperture lidar image η steering efficiency due to quantization error ηatm transmission of the atmosphere in one direction ηh heterodyne mixing efficiency ηsys total transmission of the lidar system, both in and out θ angular motion created by the lenslet θmax maximum steering angle
angle of deflection for an AO modulator
full beam width, half maximum diffraction limit λ wavelength λi wavelength of the idler laser λp wavelength of the pump laser λs wavelength of the signal laser Λ acousto-optical wavelength Λ width between resets ΛF width of the flyback region ν carrier frequency of light (ω = 2πν) ρ radius of the microlens ρt reflectance of the area σ cross section τ0 coherence time τd time required to return to no-voltage state τm mode-locked pulse separation φ phase retardation of the half-wave plate ωsig frequency (in radians) of the return signal ωLO frequency (in radians) of the local oscillator |
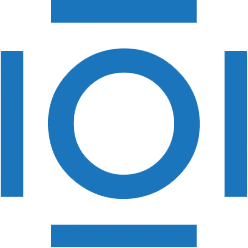
CITATIONS
LIDAR
Fiber lasers
Laser processing
Laser safety
Laser resonators
Laser scattering
Quantum cascade lasers